LASER DIODE TUNING
An Overview of Current and Temperature Tuning Coefficients
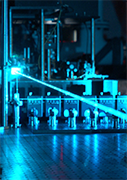
Current and Temperature Tuning of Laser Diodes
This article will help you understand the underlying principals used to derive current and/or temperature tuning coefficients.
Updated: January 15, 2021
Author: Stephen Gwinner, Laser Lab Source
INTRODUCTION:
One of the advantages of semiconductor laser diodes compared to other laser technologies is their ability to be tuned to an adjacent wavelength. An important specification for laser diode’s used in tunable diode laser absorption spectroscopy (TDLAS) is the laser's tuning coefficient. This is specified on the data sheet as picometers of change per milliamp of change in the bias current, and nanometers of change per change in degrees Celsius. More and more sensing and spectroscopic applications are emerging which require tuning the output of single mode semiconductor lasers in order to measure the abosorption level of laser light through a gas sample. This absorption level is then used to derive the concentration of the gas molecule of interest in the sample. Once the concentration level is established, monitoring and feedback control systems can be implemented. This article is an overview of the underlying principles of the tuning coefficient, also known as the tuning derivative.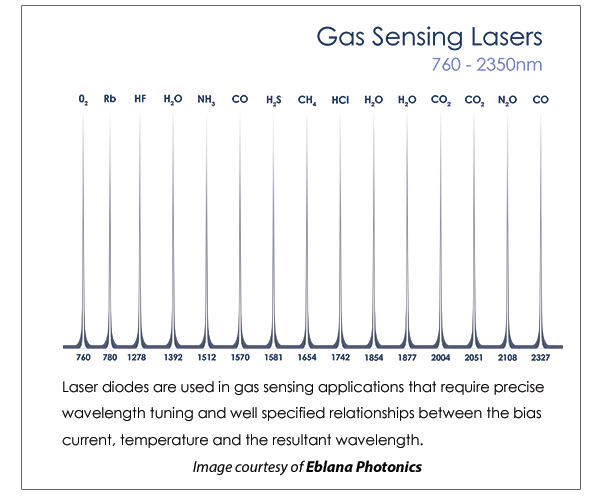
gas sensing laser diode wavelengths
APPLICATIONS WHICH REQUIRE WELL QUANTIFIED WAVELENGTH TUNING COEFFICIENTS (pm/mA and nm/°C):
Laser diode sources are used in a wide array of sensing applications that require tuning. DFB lasers are commonly used for absorption based sensing of gases such as carbon dioxide, ammonia, methane and hydrogen fluoride. Single mode and single frequency lasers are also used in applications which require precise tuning and control of the frequency of the source such flourescence for in vivo cancer imaging. NIR flourescence uses a near infrared source to excite a species, which in turn will emit a specific frequency of light at a longer wavelength than the original wavelength which was used to excite the species. You can read more about non-invasive fluorescence imaging using a modulated ICCD and laser diode in this article on OSA.org »PHYSICAL CHANGES TO A LASER DIODE FROM VARYING TEMPERATURE AND INJECTED CURRENT DENSITY:
The lasing wavelength of a laser diode is typically controlled by varying the temperature and the injected drive current. The physical changes to the laser diode resulting from varying these two control parameters are listed below and shown in Figure [1].
- Bandgap energy changes due to changes in temperature, and a band-filling effect induced by drive current injected into the active layer of the semiconductor.
- A change to the refractive index in the active layer, which is induced by changes in ambient temperature and temperature changes resulting from the Joule heating associated with injected drive current density.

laser diode tuning properties
MEASURING CHANGES IN WAVELENGTH TO DERIVE LASER DIODE TUNING COEFFICIENTS:
The change in a laser diode’s lasing wavelength is primarily a result of a temperature change in the active layer, also known as the pn-junction temperature or simply the junction temperature. This temperature change is mainly the result of controlling ambient device temperature and the injected drive current. Measuring the change in lasing wavelength allows us to analyze the effects of changing ambient temperature and injected drive current which induce the physical changes referred to above. Subsequently, we are able to derive the temperature and current tuning coefficients (pm/mA and nm/°C). To see tuning in practice, here is a short video which shows the affect of tuning the temperature and the current density on the wavelength:temperature tuning a laser diode video
INJECTED CURRENT DENSITY (DRIVE CURRENT) CHANGES AND EFFECTS ON LASER DIODE WAVELENGTH:
The rate of temperature change in the active layer is produced by the Joule heating associated with injected current density. The rate is strongly affected by heat conduction. The heat generated in the active layer diffuses from the active layer to the surrounding regions. The resultant rate of change in wavelength is strongly impacted by the laser diodes mounting configuration and packaging structure as shown in figure [2].![laser diode heat sinking/>
<p><em>heat sinking a laser diode</em></p>
<br />
<p><strong>AMBIENT TEMPERATURE CHANGES AND EFFECTS ON WAVELENGTH:</strong><br />Heat is transmitted to, or removed from the active layer of the laser diode when the environmental temperature changes or the devices package temperature is actively changed. The heat conductance of the package, package stem, and heat sink is the same as the diffusion of Joule heating at the active layer shown in figure [2] above. A gas absorption spectrum is shown below in figure [4] to illustrate changes in ambient temperature. The laser diode was biased at 60 mA and the ambient temperature was changed in 0.2°C increments.</p>
<img src=](/com.laserdiodesource/images/laser-diode-tuning-2.png)
effect of increasing heat on a laser diode
CONCLUSION:
The tuning coefficients stated on a laser diode’s data sheet are primarily derived from inducing temperature changes in the active layer of the semiconductor and monitoring resultant wavelength changes. Tuning coefficients are listed on laser diode data sheets as pm/mA and/or nm/°C. Tuning coefficients will vary with the scanning rate used to monitor the changes in wavelength associated with changes to ambient temperature or injected current. The scanning rate is typically strictly fixed to eliminate monitoring errors. Also, while not discussed in this article, it is important to note that since the change in lasing wavelength is primarily caused by a change in junction temperature, it is common to first establish a baseline “known” junction temperature at a given current and temperature control set point. This is typically a measurement of changes to junction voltage and thermal resistance related to changing injection current.References:
- Carl Hepburn. Britneys guide to semiconductor physics. http://www.britneyspears.ac/lasers.htm.
- Nagaatsu Ogasawara. Lasers, semiconductor. Technical report, Nagaatsu Ogasawara University of Electro-Communications, www.pro-physik.de/Phy/pdfs/ OE042_1.pdf.
- M. Fukuda, T. Mishima, N. Nakayama, and T. Masuda,. Appl Phys B. Vol.100. 2010.
- Sigrist MW, editor. Appl Phys B. 2. Vol. 90. 2008.
- Fukuda M. Optical Semiconductor Devices. Wiley; New York: 1999.
- Nash FR. J Appl Phys. 1973;44:4696.
- Yamamoto Y. IEEE J Quantum Electron. 1983;QE-19:34.